Skeletal fragility in type 2 diabetes: assessment and management
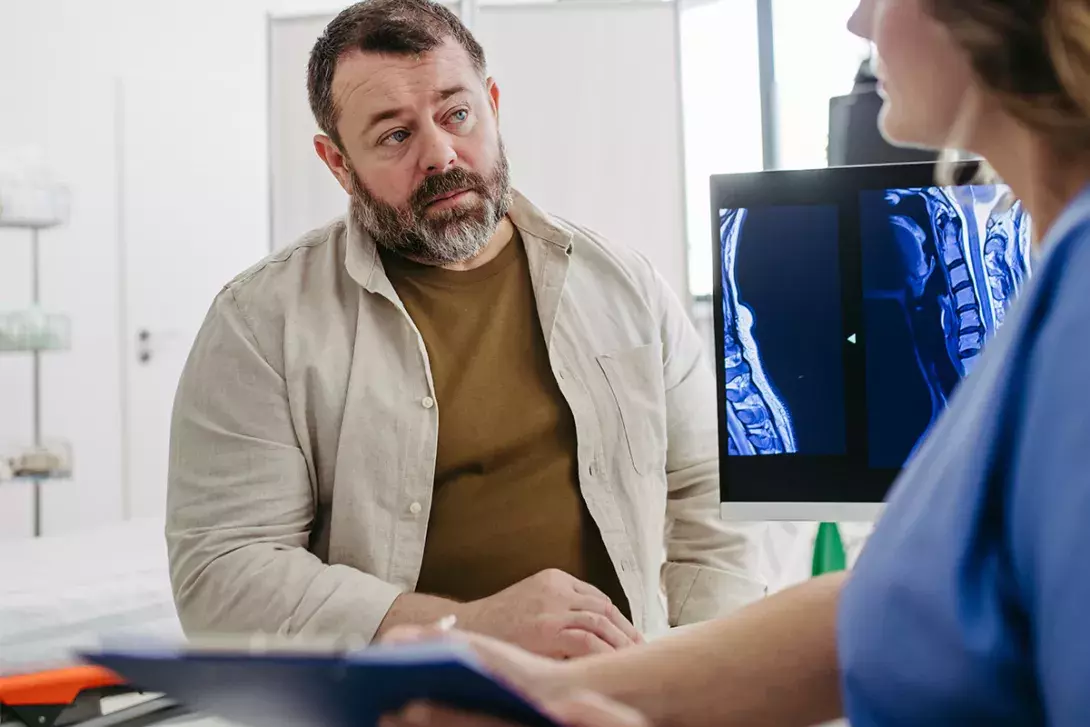
Skeletal fragility is increasingly recognised as a complication of diabetes, with patients with type 2 diabetes at a higher risk of some fragility fractures. Extra considerations are required during the calculation of fracture risk for these patients, as well as for the selection of the most suitable investigations and treatments.
- Patients with type 2 diabetes mellitus (T2DM) have an increased risk of fracture despite relatively preserved bone mineral density on dual-energy x-ray absorptiometry.
- The underlying metabolic contributors to skeletal fragility in T2DM are complex and include obesity, hyperinsulinaemia, hyperglycaemia and advanced glycation end products.
- The hallmarks of diabetic osteopathy are impaired bone microarchitecture and low bone turnover, both of which increase fracture susceptibility.
- Alternative imaging modalities, including trabecular bone score and quantitative CT, allow for noninvasive assessment of bone microarchitecture and provide insight into skeletal fragility.
- Fracture risk calculators underestimate fracture risk in T2DM; adjustments can be made to the standard fracture risk calculators to improve risk prediction.
- T2DM medications have differing effects on fracture risk.
- Currently available osteoporosis treatments are safe and effective in T2DM.
Patients with type 2 diabetes mellitus (T2DM) are at increased risk of fracture and fracture-associated consequences. The underlying metabolic contributors to skeletal fragility in T2DM are complex and include obesity, hyperinsulinaemia, hyperglycaemia and certain diabetes medications. Bone mineral density (BMD) is usually relatively preserved when measured by dual-energy x-ray absorptiometry (DXA) and alternative imaging modalities may provide greater assessment of skeletal fragility. Current fracture risk calculators underestimate fracture risk in patients with T2DM and commencement of osteoporosis-specific therapy may be overlooked. Adjusted fracture risk calculators and treatment thresholds should consider T2DM to ensure this vulnerable group of patients receives timely and appropriate skeletal management.
The burden of skeletal fragility in T2DM
T2DM is associated with poor bone health, resulting in increased risk of some fractures, prolonged post-fracture recovery and increased post-fracture mortality. Meta-analyses of fracture risk in T2DM have shown an increased risk of any fracture (relative risk [RR], 1.2), particularly at the hip (RR, 1.3-2.1), even when controlled for age, body mass index (BMI) and physical activity.1-4 The risk of fractures of the ribs, humerus, distal leg and ankle are also elevated.5,6 The risk of vertebral fractures in T2DM is unclear, owing partly to the difficulty in ascertaining the risk of vertebral fractures across different studies. Given many vertebral fractures are asymptomatic and are detected incidentally, vstudies that do not use systematic routine radiography may underestimate the true incidence of vertebral fractures. Therefore, the risk of vertebral fractures in T2DM subjects could be overestimated (relative to control subjects), as they are more likely to present to healthcare professionals than the general population.
Fracture risk in T2DM varies according to diabetes-related characteristics. Differences in observed fracture risk appears to be related, in part, to the clinical heterogeneity of the studied populations (Figure 1 ). A Swedish national registry of adult patients with T2DM showed a marginally increased risk of major osteoporotic fractures (defined as a composite of hip, clinical spine, distal forearm and proximal humerus) (hazard ratio [HR], 1.01; 95% confidence interval [CI], 1.00 to 1.03) and hip fractures (HR, 1.06; 95% CI, 1.04 to 1.08) compared with controls. However, the presence of one of four risk factors, such as low BMI, longer duration of T2DM, insulin treatment or low physical activity, resulted in a substantial increase (more than 20%; HR, 1.20) in fracture risk.5 A post hoc analysis of the randomised controlled Fenofibrate Intervention and Event Lowering in Diabetes (FIELD) study found insulin therapy and sex-specific vascular complications were associated with an increased risk of any fracture in T2DM.7 An increased risk of fracture has also been noted in patients with a longer duration of diabetes and extremes of glycaemic control, with glycated haemoglobin (HbA1c) levels of below 6.5% and higher than 9.5% conferring a higher risk of fracture.5,8,9 Postulated mechanisms for this include the direct effects on bone microarchitecture (as further detailed below) and hypoglycaemia-related falls. Poorer functional status, including recurrent falls and frailty, has also been associated with increased fractures.10,11 However, the data is confounded by a number of variables, since many of these factors often coexist in patients. For example, patients with a longer duration of T2DM or worse glycaemic control are more likely to have complications and use certain types of medications. Therefore, the independent effects of these factors are difficult to determine in the absence of prospective trials that simultaneously collect detailed metabolic and bone characteristics.
Importantly, people with T2DM suffer worse post-fracture outcomes, including more deep wound infections, delayed wound healing, reduced functionality and an increased 30-day mortality risk post-fracture.12,13 Longer-term mortality is also increased, with a 30 to 50% increase in mortality following any fracture, including fractures at nonhip and nonvertebral sites.14-16
Pathophysiology of skeletal fragility in T2DM
The underlying metabolic contributors to skeletal fragility in T2DM are complex and include obesity, hyperinsulinaemia, hyperglycaemia and advanced glycation end products (AGEs) (Figure 1).17 Each of these factors may have opposing skeletal effects, with various degrees of relative importance as the patient’s T2DM progresses. Obesity leads to mechanical loading on the skeleton, which, together with hyperinsulinaemia, usually improves BMD. However, chronic inflammation with proinflammatory cytokines and adipokines may negatively affect bone health and increase fracture risk. Hyperglycaemia directly impairs bone formation and bone structure, leading to accelerated bone demineralisation, impaired mechanosensing and poorer resistance to stress.18,19 Chronic hyperglycaemia and accumulation of AGEs are associated with the development of diabetes-related vascular complications through oxidative stress and accelerated ageing. In bone, the deposition of AGEs disrupts bone mineralisation, increases bone resorption and inhibits bone formation.20,21 Thus, potential anabolic effects from mechanical loading and hyperinsulinaemia may be offset by impaired bone structure and turnover, leading to reduced strength loading and increased skeletal fragility.
Assessment of skeletal fragility in T2DM
Clinically, the assessment of the skeleton in T2DM remains challenging.22 The hallmarks of diabetic osteopathy are impaired bone microarchitecture and low bone turnover, resulting in fracture susceptibility even with relatively preserved BMD. When measured by DXA, the clinical standard for measuring BMD, BMD is elevated in T2DM, owing to the often-concomitant higher BMI in patients with T2DM.23-25 As such, fracture risk in T2DM is often underestimated by BMD.26 Instead, alternative imaging modalities provide insight into the aberrations in bone microarchitecture that contribute to skeletal fragility in T2DM beyond BMD. However, the availability of these imaging modalities is largely limited to research settings and clinical validation is required.
The trabecular bone score (TBS) uses standard lumbar spine DXA imaging to assess vertebral spine microarchitecture. TBS may be reported on a DXA BMD report and can be used to estimate absolute fracture risk; however, it is not available at all centres. T2DM is associated with a lower TBS, indicative of poorer microarchitecture, and may be associated with increased fracture risk, independent of BMD.27-29
Quantitative CT (QCT) uses high resolution CT scans of the vertebrae and hip, and measures the volumetric BMD, bone structure and composition from three-dimensional reconstructions of the bone. Differentiation between cortical and trabecular bone allows a noninvasive assessment of bone microarchitecture. However, high radiation exposure precludes routine use and normative data is lacking. Nevertheless, QCT may be particularly useful in patients where DXA results are uninformative (e.g. patients with degenerative spinal disease, kyphoscoliosis) or in patients whose BMD scores by DXA are relatively preserved despite recurrent fractures. QCT is increasingly available at some radiology centres and is reimbursed by Medicare for BMD testing. Figure 2 shows DXA, TBS and QCT in a patient with T2DM and recurrent fractures, and the additional information the various modalities provide. Despite relatively preserved BMD and mild microarchitecture degradation on TBS, the patient had markedly reduced volumetric BMD on QCT, which confirmed skeletal deficits that may have explained the recurrent fractures.
High resolution peripheral quantitative QCT is limited to the distal radius and femur, and allows for precise evaluation of cortical and trabecular microarchitectural analysis with minimal radiation exposure, but its use is currently limited to research settings and it is not widely available. T2DM is associated with high cortical porosity but preserved trabecular bone, with microvascular complications and peripheral vascular disease correlating with cortical deficits.30-33 A recent meta-analysis of high resolution peripheral quantitative QCT in patients with T2DM showed increased cortical porosity with preserved trabecular parameters and increased cortical thickness that was more pronounced at the radius compared with the tibia, suggesting that mechanical loading may have a role in affecting cortical parameters.34
T2DM is a low bone turnover state.25,35,36 In normal homeostasis, bone remodelling occurs via a coupled process of bone resorption and formation. When this process is uncoupled (high or low bone turnover), bone microarchitecture is disrupted and skeletal fragility ensues.37 Procollagen type 1 N-terminal propeptide and C-telopeptide are serum bone turnover markers that reflect bone formation and resorption, respectively, and may be used as clinical surrogates for assessing bone turnover. Despite consistently lower levels of these markers in patients with T2DM, their role in predicting fracture risk in this population is unclear.38
Fracture risk calculators underestimate fracture risk in T2DM
Fracture risk calculators have been developed to evaluate an individual’s fracture risk. The Fracture Risk Assessment Tool (FRAX) and Garvan Fracture Risk Calculator use several clinical risk factors, with or without DXA-determined BMD. These algorithms provide a 5- and 10-year probability of hip and major osteoporotic fracture. However, they do not consider bone microarchitecture and consequently underestimate fracture risk in patients with T2DM. The only freely available fracture risk calculator that specifically includes T2DM as a clinical variable is QFracture, although its use is limited outside the UK.39 A recent update to FRAX, FRAXplus, allows for modification of the traditional FRAX estimate by including T2DM and TBS as risk adjustments; however, this is currently a paid addition to the standard FRAX calculator.
To improve fracture risk prediction in patients with T2DM, a number of adjustments can be made to FRAX. Fracture risk in patients with T2DM can be more accurately reflected using any of the following strategies:
- reducing the BMD T-score by 0.5 standard deviations
- adding 10 years of age
- including a patient’s TBS
- using rheumatoid arthritis as a substitute for T2DM.
However, none of these adjustments fully capture fracture risk in T2DM.26,40
A number of working groups have developed algorithms that include diabetes-related clinical factors and lower BMD thresholds for osteoporosis screening and initiation of therapy.41,42 We have updated and expanded on these management principles in two recent review articles (Figure 3 ).43,44 Additional imaging (e.g. TBS or QCT) should be considered if the clinical presentation is discordant with DXA-derived BMD scores (e.g. in a patient with a minimal trauma fracture despite normal BMD scores). However, current Medicare and PBS guidelines do not include T2DM as a secondary cause of osteoporosis for reimbursement of BMD monitoring or the initiation of osteoporosis-specific therapy. Nevertheless, active surveillance and targeted therapy may still be warranted, especially in patients with established skeletal fragility (low BMD score or history of a fragility fracture).
Effect of T2DM medications on bone
Given the plethora of non-glycaemic effects of T2DM medications, agents without negative bone effects should be preferred in those at higher skeletal risk (Table ).45-60 Although there are no prospective trial data examining bone outcomes with T2DM medications, observational data and post hoc analyses provide evidence to suggest the relative safety of some agents. Additionally, HbA1c targets to minimise falls and hypoglycaemia should be individualised.
Metformin and sulfonylureas have an overall positive or neutral effect on BMD and fracture risk.45,56,61 The impact of dipeptidyl peptidase-4 inhibitors on fracture risk has mostly been positive, with overall fracture risk reduction in meta-analyses and large cohort studies.46,47,62-64 Glucagon-like peptide-1 receptor agonists have shown positive effects on bone turnover.48,52,65,66 In one small clinical study, liraglutide protected against weight loss-associated bone loss.67
Insulin therapy has consistently been associated with an increased risk of fractures.9,56,60 The proposed mechanism includes hypoglycaemia-related falls, particularly in older people, although further studies are required to ascertain any direct skeletal effects. Thiazolidinediones have an overall negative effect on bone and have been associated with an increased risk of fracture.57,58,68,69
Early data on canagliflozin, a sodium-glucose cotransporter-2 inhibitor, raised concerns because of an increase in lower limb amputations and fractures in the landmark CANVAS trial.59 Further studies, including the CREDENCE trial, using canagliflozin, as well as dapagliflozin and empagliflozin trials, have shown no difference in fracture rates with any sodium-glucose cotransporter-2 inhibitor.53,54,70,71 Given fracture risk is greatest in patients with vascular complications, and these agents may be preferentially chosen for their renal and cardiovascular benefits, canagliflozin should be avoided in patients at higher skeletal risk until further data is available.
Finally, significant weight loss, as seen with bariatric surgery and caloric restriction, is associated with persistent bone loss and increased fractures.72-74 Monitoring of bone health and micronutrients is therefore critical in these patients. The effect on BMD and fracture risk with weight loss medications, including semaglutide and tirzepatide, have not been determined, but it is likely that these patients would benefit from closer monitoring, particularly if there are concomitant risk factors for fractures.
Bone treatment options in T2DM
Patients with T2DM have higher fracture risk with increased post-fracture mortality. Therefore, targeted bone management is warranted following any fragility fracture. The unique skeletal profile of preserved BMD and low bone turnover raises questions about the optimal management of skeletal health in patients with T2DM. There are no dedicated prospective randomised controlled trials (RCTs) examining the safety and efficacy of osteoporosis therapies in patients with T2DM, although post hoc analyses suggest their efficacy in patients with T2DM is comparable to patients without T2DM. There is no evidence for an increase in adverse effects, such as atypical femoral fractures or osteonecrosis of the jaw, in patients with T2DM using antiresorptive agents; however, on-trial events are rare overall. Muscle strengthening and physical strength should be managed through individualised supervised exercise programs to reduce falls and frailty.
Optimising calcium intake and vitamin D levels is essential for bone health. The recommended daily dietary calcium intake is 1300 mg for women older than 50 years, men older than 70 years and patients taking osteoporosis therapy; or 1000 mg for women younger than 50 years and men younger than 70 years. Ideally, this is achieved through dietary measures: three serves of dairy per day usually provides enough dietary calcium to meet the recommended dietary requirements. However, if this is not achievable, consideration can be given to a calcium supplement in the form of calcium carbonate or calcium citrate (the latter being preferable in patients using a proton pump inhibitor). A target serum 25-OH vitamin D level of at least 50 nmol/L, particularly in patients treated with antiresorptive therapy, is reasonable and should be achieved with cholecalciferol supplementation if required, keeping in mind that obese patients are more likely to require higher doses of supplementation to meet targets because of the larger volume of distribution. There is no evidence to support the use of supplemental boron or vitamin K in the treatment of osteoporosis.
Studies of the oral bisphosphonates alendronate and risedronate have found equivalent gains in BMD in individuals with T2DM compared with individuals without.75,76 In a recent meta-analysis of 15 RCTs using antiresorptive agents (including alendronate, risedronate and intravenous zoledronic acid), bisphosphonate use was associated with improved BMD measurements and a reduction in vertebral and nonvertebral fractures.77 However, the use of bisphosphonates, particularly intravenous zoledronic acid, needs to be carefully considered in patients with renal impairment.
Denosumab is increasingly used because of its convenience, relative lack of adverse effects and safety in mild to moderate renal impairment. The FREEDOM and extension trials showed comparable BMD gains and reduction in vertebral fractures in patients with and without T2DM.78 Unexpectedly, there was an increase in nonvertebral fractures with denosumab compared with placebo in participants with T2DM. It is unclear whether this is a true medication effect or the result of a low number of fractures in the placebo arm. As with the general population, care should be given when initiating denosumab in younger patients. Therapy may be indicated for a short duration to reduce imminent fracture risk (i.e. within the first three to five years of a fracture) and then ceased to prevent prolonged continuous bone turnover that is associated with adverse effects. As patients with T2DM are more likely to have higher levels of BMD, a treatment break may be warranted, and therefore the challenges of ceasing denosumab (the risk of rapid bone loss and multiple vertebral fractures with cessation of therapy) should be considered prior to denosumab initiation. Discontinuation of denosumab requires careful transition to an alternative osteoporosis therapy and should only be done under the guidance of a specialist, after consideration of the risks and benefits of doing so. There are also preliminary data that denosumab may improve glycaemic control, with a case-control study that showed significant improvement in HbA1c levels and BMI at 6 and 12 months in patients with T2DM and prediabetes treated with denosumab, compared with calcium and vitamin D or bisphosphonate.79
Menopausal hormone therapy provides an alternative option for women under the age of 60 years who are within 10 years of menopause, although the overall cardiovascular and breast cancer risk, both of which are inherently increased in T2DM, must be considered. Raloxifene has shown a reduction in fractures in women with and without T2DM.80-82 There are no specific data on the use of tibolone in T2DM.
Considering the low bone turnover state in T2DM, anabolic agents (teriparatide and romosozumab) have a theoretical benefit over antiresorptive agents. Anabolic agents require initiation by a consultant physician and patients should be managed in a specialist setting. Anabolic agents in diabetic mice models showed restoration of bone microarchitecture and improvement in cortical porosity and mechanical properties, demonstrating a therapeutic value of anabolic agents to specifically address the underlying pathophysiology of diabetic osteopathy.83
Teriparatide is a recombinant fragment of parathyroid hormone (PTH) 1-34 and is given as a daily subcutaneous injection for up to 24 months, followed by consolidation with antiresorptive therapy. Unlike chronic exposure to PTH, which results in increased bone resorption and bone loss, intermittent PTH exposure stimulates bone formation. Teriparatide showed similar improvement in BMD and vertebral fracture reduction in subjects with and without T2DM in a post hoc analysis of an observational study (DANCE).84 From a subsequent pooled analysis, which included three further observational studies, teriparatide use in individuals with T2DM was associated with a greater reduction in all clinical fractures compared with subjects without T2DM.84
Romosozumab is a humanised monoclonal antibody that targets and inhibits the protein sclerostin, preventing the inhibition of bone formation through the Wnt signalling pathway, leading to bone formation and substantial BMD gains. Romosozumab is one of the most potent anabolic agents available and was approved for PBS-subsidised use in Australia for severe osteoporosis in April 2022. It is given as a monthly subcutaneous injection for 12 months and should be followed by timely consolidation with antiresorptive therapy to lock in bone density gains. There are no data on the safety or efficacy of romosozumab in patients with T2DM; the potential increase in cardiovascular events, seen in the alendronate-controlled (ARCH) trial but not in the placebo-controlled RCT (FRAME), warrants further investigation.85,86 Currently, romosozumab is contraindicated in Australia in patients with a history of stroke or myocardial infarction.
Conclusion
T2DM is associated with an increased risk of fractures and post-fracture adverse sequelae. However, treatment is often overlooked when using standard fracture risk calculators because of the relatively preserved BMD in patients with T2DM and a lack of clinical guidelines for diabetic osteopathy. Impaired bone microarchitecture and low bone turnover orchestrated by underlying pathophysiological drivers may not be reflected by BMD scores using DXA; alternative imaging modalities such as TBS and QCT may be employed to better assess skeletal deficits. Fracture risk calculators that incorporate diabetes-related factors and adjustments for T2DM may identify those at increased fracture risk. Treatment thresholds should be adjusted, given the significance of fracture-associated morbidity and mortality. Although prospective RCTs specific to patients with T2DM are lacking, currently available bone therapies are probably safe and effective in T2DM, and should be considered for patients with established skeletal fragility to ensure this vulnerable population receives adequate treatment. MT
COMPETING INTERESTS: None.
References
1. Fan Y, Wei F, Lang Y, Liu Y. Diabetes mellitus and risk of hip fractures: a meta-analysis. Osteoporos Int 2016; 27: 219-228.
2. Dytfeld J, Michalak M. Type 2 diabetes and risk of low-energy fractures in postmenopausal women: meta-analysis of observational studies. Aging Clin Exp Res 2017; 29: 301-309.
3. Janghorbani M, Van Dam RM, Willett WC, Hu FB. Systematic review of type 1 and type 2 diabetes mellitus and risk of fracture. Am J Epidemiol 2007; 166: 495-505.
4. Vestergaard P. Discrepancies in bone mineral density and fracture risk in patients with type 1 and type 2 diabetes – a meta-analysis. Osteoporos Int 2007; 18: 427-444.
5. Axelsson KF, Litsne H, Kousoula K, Franzén S, Eliasson B, Lorentzon M. Risk of fracture in adults with type 2 diabetes in Sweden: a national cohort study. PLoS Med 2023; 20: e1004172.
6. Bonds DE, Larson JC, Schwartz AV, et al. Risk of fracture in women with type 2 diabetes: the Women’s Health Initiative Observational Study. J Clin Endocrinol Metab 2006; 91: 3404-3410.
7. Sheu A, O’Connell RL, Jenkins AJ, et al. Factors associated with fragility fractures in type 2 diabetes: an analysis of the randomised controlled Fenofibrate Intervention and Event Lowering in Diabetes (FIELD) study. Diabetes Metab Res Rev 2023: e3631.
8. Lee RH, Sloane R, Pieper C, et al. Glycemic control and insulin treatment alter fracture risk in older men with type 2 diabetes mellitus. J Bone Miner Res 2019; 34: 2045-2051.
9. Ivers RQ, Cumming RG, Mitchell P, Peduto AJ. Diabetes and risk of fracture: the Blue Mountains Eye Study. Diabetes Care 2001; 24: 1198-1203.
10. Li G, Prior JC, Leslie WD, et al. Frailty and risk of fractures in patients with type 2 diabetes. Diabetes Care 2019; 42: 507-513.
11. Dufour AB, Kiel DP, Williams SA, Weiss RJ, Samelson EJ. Risk factors for incident fracture in older adults with type 2 diabetes: the Framingham Heart Study. Diabetes Care 2021; 44: 1547-1555.
12. Marin C, Luyten FP, Van der Schueren B, Kerckhofs G, Vandamme K. The impact of type 2 diabetes on bone fracture healing. Frontiers in Endocrinology Front Endocrinol 2018; 9: 6.
13. Liao C-C, Lin C-S, Shih C-C, et al. Increased risk of fracture and postfracture adverse events in patients with diabetes: two nationwide population-based retrospective cohort studies. Diabetes Care 2014; 37: 2246-2252.
14. Madsen CM, Jantzen C, Norring-Agerskov D, et al. Excess mortality following hip fracture in patients with diabetes according to age: a nationwide population-based cohort study of 154,047 hip fracture patients. Age Ageing 2019; 48: 559-563.
15. Martinez-Laguna D, Nogues X, Abrahamsen B, et al. Excess of all-cause mortality after a fracture in type 2 diabetic patients: a population-based cohort study. Osteoporos Int 2017; 28: 2573-2581.
16. Sheu A, Bliuc D, Tran T, White CP, Center JR. Fractures in type 2 diabetes confer excess mortality: The Dubbo Osteoporosis Epidemiology Study. Bone 2022; 159: 116373.
17. Sheu A, Greenfield JR, White CP, Center JR. Contributors to impaired bone health in type 2 diabetes. Trends Endocrinol Metab 2023; 34: 34-48.
18. Eckhardt BA, Rowsey JL, Thicke BS, et al. Accelerated osteocyte senescence and skeletal fragility in mice with type 2 diabetes. JCI Insight 2020; 5: e135236.
19. Wang A, Midura RJ, Vasanji A, Wang AJ, Hascall VC. Hyperglycemia diverts dividing osteoblastic precursor cells to an adipogenic pathway and induces synthesis of a hyaluronan matrix that is adhesive for monocytes. J Biol Chem 2014; 289: 11410-11420.
20. Napoli N, Chandran M, Pierroz DD, et al. Mechanisms of diabetes mellitus-induced bone fragility. Nat Rev Endocrinol 2017; 13: 208-219.
21. Hein GE. Glycation endproducts in osteoporosis – is there a pathophysiologic importance? Clin Chim Acta 2006; 371: 32-36.
22. Sheu A, Greenfield JR, White CP, Center JR. Assessment and treatment of osteoporosis and fractures in type 2 diabetes. Trends Endocrinol Metab 2022; 33: 333-344.
23. Ma L, Oei L, Jiang L, et al. Association between bone mineral density and type 2 diabetes mellitus: a meta-analysis of observational studies. Eur J Epidemiol 2012; 27: 319-332.
24. Hofbauer LC, Brueck CC, Singh SK, Dobnig H. Osteoporosis in patients with diabetes mellitus. J Bone Min Res 2007; 22: 1317-1328.
25. Sheu A, Blank RD, Tran T, et al. Associations of type 2 diabetes, body composition, and insulin resistance with bone parameters: the Dubbo Osteoporosis Epidemiology Study. JBMR Plus 2023; 7: e10780.
26. Schwartz AV, Vittinghoff E, Bauer DC, et al. Association of BMD and FRAX score with risk of fracture in older adults with type 2 diabetes. JAMA 2011; 305: 2184-2192.
27. Dhaliwal R, Cibula D, Ghosh C, Weinstock R, Moses A. Bone quality assessment in type 2 diabetes mellitus. Osteoporos Int 2014; 25: 1969-1973.
28. Kim JH, Choi HJ, Ku EJ, et al. Trabecular bone score as an indicator for skeletal deterioration in diabetes. J Clin Endocrinol Metab 2015; 100: 475-482.
29. Leslie WD, Aubry-Rozier B, Lamy O, Hans D, Program MBD. TBS (trabecular bone score) and diabetes-related fracture risk. J Clin Endocrinol Metab 2013; 98: 602-609.
30. Burghardt AJ, Issever AS, Schwartz AV, et al. High-resolution peripheral quantitative computed tomographic imaging of cortical and trabecular bone microarchitecture in patients with type 2 diabetes mellitus. J Clin Endocrinol Metab 2010; 95: 5045-5055.
31. Samelson EJ, Demissie S, Cupples LA, et al. Diabetes and deficits in cortical bone density, microarchitecture, and bone size: Framingham HR‐pQCT study. J Bone Min Res 2018; 33: 54-62.
32. Shanbhogue VV, Hansen S, Frost M, et al. Compromised cortical bone compartment in type 2 diabetes mellitus patients with microvascular disease. Eur J Endocrinol 2016; 174: 115-124.
33. Samakkarnthai P, Sfeir JG, Atkinson EJ, et al. Determinants of bone material strength and cortical porosity in patients with type 2 diabetes mellitus. J Clin Endocrinol Metab 2020; 105: e3718-e29.
34. Walle M, Whittier DE, Frost M, Müller R, Collins CJ. Meta-analysis of diabetes mellitus-associated differences in bone structure assessed by high-resolution peripheral quantitative computed tomography. Curr Osteoporos Rep 2022; 20: 398-409.
35. Starup-Linde J, Eriksen S, Lykkeboe S, Handberg A, Vestergaard P. Biochemical markers of bone turnover in diabetes patients – a meta-analysis, and a methodological study on the effects of glucose on bone markers. Osteoporos Int 2014; 25: 1697-1708.
36. Starup-Linde J, Vestergaard P. Biochemical bone turnover markers in diabetes mellitus – a systematic review. Bone 2016; 82: 69-78.
37. Johnell O, Odén A, De Laet C, Garnero P, Delmas P, Kanis J. Biochemical indices of bone turnover and the assessment of fracture probability. Osteoporos Int 2002; 13: 523-526.
38. Napoli N, Conte C, Eastell R, et al. Bone turnover markers do not predict fracture risk in type 2 diabetes. J Bone Min Res 2020; 35: 2363-2371.
39. Hippisley-Cox J, Coupland C. Derivation and validation of updated QFracture algorithm to predict risk of osteoporotic fracture in primary care in the United Kingdom: prospective open cohort study. BMJ 2012; 344: e3427.
40. Leslie WD, Johansson H, McCloskey EV, Harvey NC, Kanis JA, Hans D. Comparison of methods for improving fracture risk assessment in diabetes: the Manitoba BMD Registry. J Bone Miner Res 2018; 33: 1923-1930.
41. Ferrari SL, Abrahamsen B, Napoli N, et al. Diagnosis and management of bone fragility in diabetes: an emerging challenge. Osteoporos Int 2018; 29: 2585-2596.
42. Chiodini I, Gaudio A, Palermo A, et al. Management of bone fragility in type 2 diabetes: perspective from an interdisciplinary expert panel. Nutr Metab Cardiovasc Dis 2021; 31: 2210-2233.
43. Sheu A, White CP, Center JR. Bone metabolism in diabetes: a clinician’s guide to understanding the bone–glucose interplay. Diabetologia 2024: 1-14.
44. Forner P, Sheu A. Bone health in patients with type 2 diabetes. J Endocr Soc 2024; 8: bvae112.
45. Vestergaard P, Rejnmark L, Mosekilde L. Relative fracture risk in patients with diabetes mellitus, and the impact of insulin and oral antidiabetic medication on relative fracture risk. Diabetologia 2005; 48: 1292-1299.
46. Monami M, Dicembrini I, Antenore A, Mannucci E. Dipeptidyl peptidase-4 inhibitors and bone fractures: a meta-analysis of randomized clinical trials. Diabetes Care 2011; 34: 2474-2476.
47. Yang J, Huang C, Wu S, et al. The effects of dipeptidyl peptidase-4 inhibitors on bone fracture among patients with type 2 diabetes mellitus: a network meta-analysis of randomized controlled trials. PLoS One 2017; 12: e0187537.
48. Zhang Y, Weng W, Xie B, et al. Glucagon-like peptide-1 receptor agonists and fracture risk: a network meta-analysis of randomized clinical trials. Osteoporos Int 2018; 29: 2639-2644.
49. Colhoun H, Livingstone S, Looker H, et al. Hospitalised hip fracture risk with rosiglitazone and pioglitazone use compared with other glucose-lowering drugs. Diabetologia 2012; 55: 2929-2937.
50. Napoli N, Schwartz AV, Schafer AL, et al. Vertebral fracture risk in diabetic elderly men: the MrOS Study. J Bone Min Res 2018; 33: 63-69.
51. Mosenzon O, Wei C, Davidson J, et al. Incidence of fractures in patients with type 2 diabetes in the SAVOR-TIMI 53 trial. Diabetes Care 2015; 38: 2142-2150.
52. Su B, Sheng H, Zhang M, et al. Risk of bone fractures associated with glucagon-like peptide-1 receptor agonists’ treatment: a meta-analysis of randomized controlled trials. Endocrine 2015; 48: 107-115.
53. Tang HL, Li D, Zhang J, et al. Lack of evidence for a harmful effect of sodium‐glucose co‐transporter 2 (SGLT2) inhibitors on fracture risk among type 2 diabetes patients: a network and cumulative meta‐analysis of randomized controlled trials. Diabetes Obes Metab 2016; 18: 1199-1206.
54. Ruanpeng D, Ungprasert P, Sangtian J, Harindhanavudhi T. Sodium‐glucose cotransporter 2 (SGLT2) inhibitors and fracture risk in patients with type 2 diabetes mellitus: a meta‐analysis. Diabetes Metab Res Rev 2017; 33: e2903.
55. Zhuo M, Hawley CE, Paik JM, et al. Association of sodium-glucose cotransporter–2 inhibitors with fracture risk in older adults with type 2 diabetes. JAMA Netw Open 2021; 4: e2130762.
56. Napoli N, Strotmeyer ES, Ensrud KE, et al. Fracture risk in diabetic elderly men: the MrOS study. Diabetologia 2014; 57: 2057-2065.
57. Grey A, Bolland M, Gamble G, et al. The peroxisome proliferator-activated receptor-γ agonist rosiglitazone decreases bone formation and bone mineral density in healthy postmenopausal women: a randomized, controlled trial. J Clin Endocrinol Metab 2007; 92: 1305-1310.
58. Zhu Z-N, Jiang Y-F, Ding T. Risk of fracture with thiazolidinediones: an updated meta-analysis of randomized clinical trials. Bone 2014; 68: 115-23.
59. Watts NB, Bilezikian JP, Usiskin K, et al. Effects of canagliflozin on fracture risk in patients with type 2 diabetes mellitus. J Clin Endocrinol 2016; 101: 157-166.
60. Nicodemus KK, Folsom AR. Type 1 and type 2 diabetes and incident hip fractures in postmenopausal women. Diabetes Care 2001; 24: 1192-1197.
61. Gilbert MP, Marre M, Holst JJ, et al. Comparison of the long-term effects of liraglutide and glimepiride monotherapy on bone mineral density in patients with type 2 diabetes. Endocr Pract 2016; 22: 406-411.
62. Choi H, Park C, Lee Y-K, Ha Y-C, Jang S, Shin C. Risk of fractures and diabetes medications: a nationwide cohort study. Osteoporos Int 2016; 27: 2709-2715.
63. Hirshberg B, Parker A, Edelberg H, Donovan M, Iqbal N. Safety of saxagliptin: events of special interest in 9156 patients with type 2 diabetes mellitus. Diabetes Metab Res Rev 2014; 30: 556-569.
64. Josse RG, Majumdar SR, Zheng Y, et al. Sitagliptin and risk of fractures in type 2 diabetes: results from the TECOS trial. Diabetes Obes Metab 2017; 19: 78-86.
65. Viggers R, Rasmussen NHh, Vestergaard P. Effects of incretin therapy on skeletal health in type 2 diabetes – a systematic review. JBMR Plus 2023; 7: e10817.
66. Ma X, Meng J, Jia M, et al. Exendin‐4, a glucagon‐like eptide‐1 receptor agonist, prevents osteopenia by promoting bone formation and suppressing bone resorption in aged ovariectomized rats. J Bone Min Res 2013; 28: 1641-1652.
67. Hygum K, Harsløf T, Jørgensen NR, Rungby J, Pedersen SB, Langdahl BL. Bone resorption is unchanged by liraglutide in type 2 diabetes patients: a randomised controlled trial. Bone 2020; 132: 115197.
68. Benvenuti S, Cellai I, Luciani P, et al. Rosiglitazone stimulates adipogenesis and decreases osteoblastogenesis in human mesenchymal stem cells. J endocrinol invest 2007; 30: RC26-RC30.
69. Loke YK, Singh S, Furberg CD. Long-term use of thiazolidinediones and fractures in type 2 diabetes: a meta-analysis. CMAJ 2009; 180: 32-39.
70. Perkovic V, Jardine MJ, Neal B, et al. Canagliflozin and renal outcomes in type 2 diabetes and nephropathy. NEJM N Engl J Med 2019; 380: 2295-2306.
71. Bolinder J, Ljunggren Ö, Johansson L, et al. Dapagliflozin maintains glycaemic control while reducing weight and body fat mass over 2 years in patients with type 2 diabetes mellitus inadequately controlled on metformin. Diabetes Obes Metab 2014; 16: 159-169.
72. Seimon RV, Wild-Taylor AL, McClintock S, et al. 3-year effect of weight loss via severe versus moderate energy restriction on body composition among postmenopausal women with obesity – the TEMPO Diet Trial. Heliyon 2020; 6: e04007.
73. Ahlin S, Peltonen M, Sjöholm K, et al. Fracture risk after three bariatric surgery procedures in Swedish obese subjects: up to 26 years follow‐up of a controlled intervention study. J Intern Med 2020; 287: 546-557.
74. Khalid SI, Omotosho PA, Spagnoli A, Torquati A. Association of bariatric surgery with risk of fracture in patients with severe obesity. JAMA Netw Open 2020; 3: e207419-e.
75. Keegan THM, Schwartz AV, Bauer DC, Sellmeyer DE, Kelsey JL. Effect of alendronate on bone mineral density and biochemical markers of bone turnover in type 2 diabetic women: the fracture intervention trial. Diabetes Care 2004; 27: 1547-1553.
76. Inoue D, Muraoka R, Okazaki R, Nishizawa Y, Sugimoto T. Efficacy and safety of risedronate in osteoporosis subjects with comorbid diabetes, hypertension, and/or dyslipidemia: a post hoc analysis of phase III trials conducted in Japan. Calcif Tissue Int 2016; 98: 114-122.
77. Eastell R, Vittinghoff E, Lui LY, et al. Diabetes mellitus and the benefit of antiresorptive therapy on fracture risk. J Bone Min Res 2020; 37: 2121-2131.
78. Ferrari S, Eastell R, Napoli N, et al. Denosumab in postmenopausal women with osteoporosis and diabetes: subgroup analysis of FREEDOM and FREEDOM extension. Bone 2020; 134: 115268.
79. Weivoda MM, Chew CK, Monroe DG, et al. Identification of osteoclast-osteoblast coupling factors in humans reveals links between bone and energy metabolism. Nat Commun 2020; 11: 87.
80. Johnell O, Kanis JA, Black DM, et al. Associations between baseline risk factors and vertebral fracture risk in the Multiple Outcomes of Raloxifene Evaluation (MORE) Study. J Bone Min Res 2004; 19: 764-772.
81. Ensrud KE, Stock JL, Barrett‐Connor E, et al. Effects of raloxifene on fracture risk in postmenopausal women: the Raloxifene Use for the Heart Trial. J Bone Min Res 2008; 23: 112-120.
82. Vestergaard P, Rejnmark L, Mosekilde L. Are antiresorptive drugs effective against fractures in patients with diabetes? Calcif Tissue Int 2011; 88: 209-214.
83. Marino S, Akel N, Li S, et al. Reversal of the diabetic bone signature with anabolic therapies in mice. Bone Res 2023; 11: 19.
84. Langdahl BL, Silverman S, Fujiwara S, et al. Real-world effectiveness of teriparatide on fracture reduction in patients with osteoporosis and comorbidities or risk factors for fractures: integrated analysis of 4 prospective observational studies. Bone 2018; 116: 58-66.
85. Saag KG, Petersen J, Brandi ML, et al. Romosozumab or alendronate for fracture prevention in women with osteoporosis. N Engl J Med NEJM 2017; 377: 1417-1427.
86. Cosman F, Crittenden DB, Ferrari S, et al. FRAME study: the foundation effect of building bone with 1 year of romosozumab leads to continued lower fracture risk after transition to denosumab. J Bone Min Res 2018; 33: 1219-1226.