Using antidepressants in general practice: is there a role for pharmacogenomic testing?
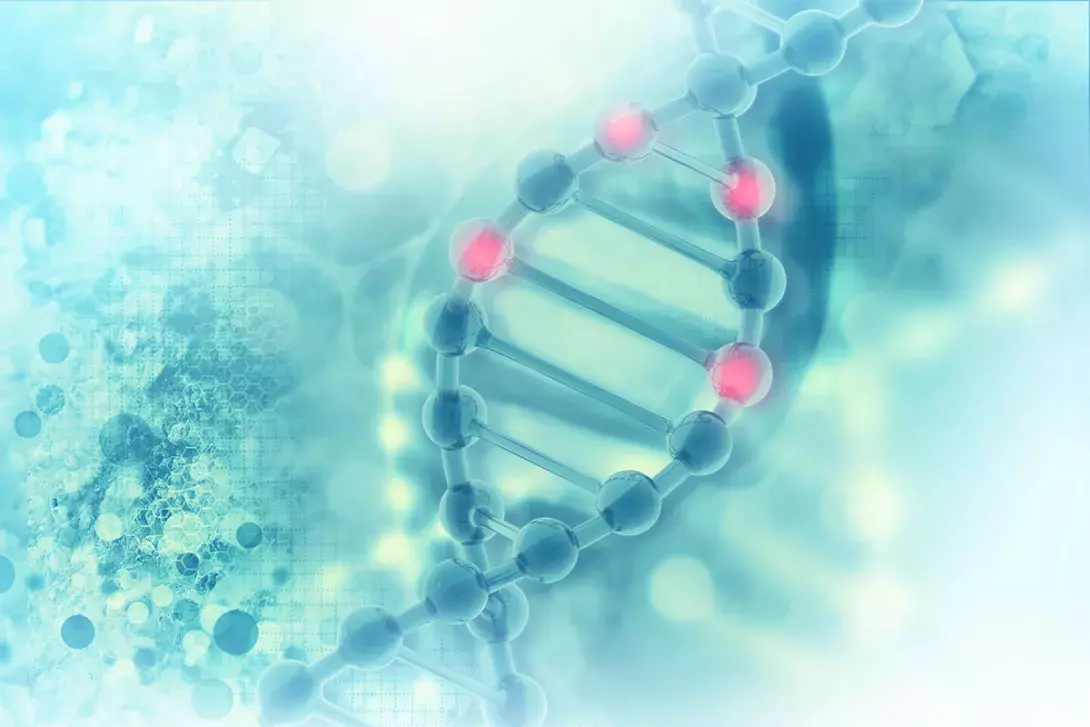
A major challenge for GPs treating patients with depression is that only one-third achieve remission with the initial antidepressant and many experience significant adverse effects. Pharmacogenomic testing for gene variants that affect antidepressant concentrations in the blood and brain has been proposed as a solution to guide antidepressant choice. However, randomised controlled trials of pharmacogenomic-guided prescribing suggest a modest benefit and therefore a limited role in routine practice.
Depression is common, with 5% of people in Australia experiencing a major depressive episode per annum.1 The impact of depression is vastly underestimated. It silently robs individuals of their enjoyment of daily life and moreover affects their capacity to engage with family and friends and contribute to their workplace and broader community.2 Most tragically, depression also increases the risk of premature mortality by either physical illness or suicide.
Depression is a frequently managed presentation in general practice. According to the BEACH survey of treatment in general practice, 12.4% of all GP encounters in 2015-16 were mental health-related, with depression being the most commonly managed mental health-related encounter, accounting for about one-third of cases.3 The most common form of GP management of mental health problems was prescription or recommendation of medications. However, depression often responds poorly to standard therapies, and patients commonly develop significant adverse effects from antidepressants.
Pharmacogenomic testing has been proposed as a possible solution to these problems. This article explores the evidence supporting pharmacogenomic testing as a guide to antidepressant choice and makes recommendations on its use in current practice.
The problem of poor response to antidepressants
The most comprehensive study of remission rates with a selective serotonin reuptake inhibitor (SSRI) followed by a structured series of treatments was the US Sequenced Treatment Alternatives to Relieve Depression (STAR*D) trial.4 This large outpatient study found that only one-third of the 3671 enrolled patients with depression underwent remission with the SSRI citalopram, with a further one-third responding after trials of up to three further treatments. One-third of patients with acute depression did not undergo remission at any point, even after receiving up to four evidence-based treatment options.
The frequent failure of depression to respond to first-choice and subsequent antidepressants is frustrating for both patients and their clinicians. At present, there are no clinical characteristics of depression that reliably predict the preferred choice of antidepressant. A proposed means of assisting clinicians in such choice is pharmacogenomic (pharmacogenetic) testing.
What is pharmacogenomic testing?
Pharmacogenomic testing investigates for variations in genes that:
- determine antidepressant concentrations in the bloodstream or brain (i.e. they affect pharmacokinetics)
- determine responsiveness to antidepressants or adverse effects (i.e. they affect pharmacodynamics).
The simple principle is that variations in these genes affect either clinical response or adverse effects.
Most of the pharmacogenomic test products that are commercially available in Australia assess a panel of genes that affect the pharmacokinetics or pharmacodynamics of antidepressants. This testing of multiple genes is termed ‘combinatorial testing’. The evidence for genes that affect blood or brain concentrations of antidepressants is currently much more robust than the evidence for genes that code for proteins with meaningful pharmacodynamic effects on either therapeutic response or proneness to adverse effects.
The genes most commonly assessed by commercial products are those that determine rates of hepatic metabolism of antidepressants via cytochrome P450 (CYP) enzymes; common examples of these enzymes include CYP2D6, CYP2C19 and CYP1A2 enzymes. For example, the gene CYP2D6 codes for the liver enzyme CYP2D6, which metabolises several antidepressants.
Different genetic variations of these genes may lead to patients being:
- poor metabolisers (leading to increased levels of the antidepressant in the bloodstream and a potentially increased likelihood of adverse effects)
- intermediate metabolisers (with metabolism that is reduced but less significantly than in poor metabolisers)
- normal or extensive metabolisers (synonymous terms for those who have normal levels of metabolism)
- ultrarapid metabolisers (leading to reduced concentrations of antidepressants, potentially lower efficacy and the need to increase doses).
Antidepressant concentrations in the brain are also affected by genes that determine the rate of transport across the blood-brain barrier. For example, some tests assess the gene ABCB1, which codes for the protein ABCB1 (ATP-binding cassette subfamily B member 1). This is an important efflux transporter that pumps compounds out through the blood-brain barrier.
Some pharmacogenomic test products include pharmacodynamic tests for genes that code for proteins within synapses, such as HTR2A (which codes for the serotonin 5-HT2A receptor), or transporter mechanisms, such as SLC6A4 (which codes for the serotonin transporter). The evidence for which proteins are responsible for clinically significant pharmacodynamic effects on therapeutic efficacy or adverse effects of antidepressants is currently poor.
Output of pharmacogenomic testing
Most commercially available pharmacogenomic test products are combinatorial. Some are transparent about which specific genes and alleles are assessed; others are not. Most products produce a simple output for clinicians that portrays, for example using ‘traffic light’ symbols or colours (red, green and amber), whether particular antidepressants:
- are suitable for standard use
- should be used with caution
- should not be used (with alternatives being suggested).
The algorithm behind such recommendations is usually opaque (i.e. a ‘black box’).
Most commercial products provide a broad range of pharmacogenomic results, with information on other medication classes such as antipsychotics and anticonvulsants.
In Australia, pharmacogenomic tests are available through a range of general pathology providers and specialist pharmacogenomic companies. The cost is generally about $190 to $200. These tests are not currently covered by Medicare.
Specific issues: safe use of citalopram
A specific safety issue relates to use of the SSRI citalopram. Citalopram has a rare risk at high doses or high blood concentrations of prolonging the QT interval, leading to the potentially fatal cardiac arrhythmia, torsades de pointes. The TGA warns against use of citalopram doses greater than 40 mg (or 20 mg in those aged over 65 years) or use of citalopram in CYP2C19 poor metabolisers. Citalopram is metabolised, like other SSRIs, mainly via the hepatic enzymes CYP2C19 and CYP2D6.
What proportion of the Australian population are CYP2C19 poor metabolisers and therefore at potential risk if prescribed citalopram? In 2022, data from the Australian Genetics of Depression Study was used to determine the rates of the various CYP2C19 alleles in 9531 individuals of European ancestry.5 Results showed that 2% had CYP2C19 gene alleles consistent with poor metabolism. Alleles were consistent with normal (‘extensive’) metabolism in 40%, slow (intermediate) metabolism in 26%, rapid metabolism in 27% and ultrarapid metabolism in 5%.
Evidence on the benefits of pharmacogenomic testing
What is the evidence supporting a role for pharmacogenetic testing in helping clinicians choose antidepressants that are more effective or better tolerated? One of the first large pharmacogenomic studies of clinical outcomes in depression was the GUIDED trial, published in 2019.6 This was a randomised, controlled, blinded multicentre study of 1167 patients with depression and an inadequate response to at least one antidepressant for the current episode. Patients were randomly allocated to either a pharmacogenomics-guided intervention in which clinicians had access to a pharmacogenomic test report to inform medication selections (‘guided- care’) or treatment as usual. Although no significant difference was found in the primary outcome measure of symptom improvement on the Hamilton Depression Rating Scale at week 8, there was some evidence of benefit in some of the secondary outcome measures. In particular, statistically significant differences were found between the pharmacogenomics-guided intervention and treatment-as-usual groups in response (26.0% vs 19.9%) and remission (15.3% vs 10.1%).
Among the more recent studies, the most important is the large PRIME Care Randomized Clinical Trial, published in 2022.7 In this trial of 1944 people with major depressive disorder who were initiating or switching treatment with an antidepressant, participants were randomly allocated to either treatment guided by pharmacogenomic testing or treatment as usual. The co-primary outcomes were the proportion of prescriptions with a predicted drug-gene interaction written in the 30 days after randomisation and remission of depressive symptoms as measured by the Patient Health Questionnaire-9. Remission was analysed as a repeated measure across 24 weeks by blinded raters. The main findings were:
- the pharmacogenomic-guided group was statistically more likely to receive a medication with a lower potential drug-gene interaction
- remission rates over 24 weeks were higher among patients whose care was guided by pharmacogenomic testing than among those in usual care but were not significantly different at week 24.
The authors concluded that provision of pharmacogenomics test results had small nonpersistent effects on symptom remission.
A recent high-quality meta-analysis of 10 randomised controlled trials of pharmacogenomics-guided prescription of antidepressants versus treatment as usual found that guided care for moderate-to-severe adult depression is more likely to result in remission and response than treatment as usual (both differences were significant).8 However, the authors raised concern about the limitations of the evidence base, including a high risk of bias and inconsistency between trials. They concluded that the evidence was consistent with modest benefits of pharmacogenomic-guided prescription of antidepressants.
An editorial accompanying the PRIME trial concluded that the overall results of all published randomised clinical trials of pharmacogenomic-guided antidepressant selection compared with usual treatment are ‘underwhelming’.9 The editorial discussed possible reasons, including a low prevalence of abnormal alleles in people of European ancestry (about 10%), who accounted for most trial participants. The prevalence of some of these alleles is substantially different in other groups, such as people of Asian ancestry.9 The editorial also commented on the lack of evidence for a role for the ‘pharmacodynamic’ genes included in a number of pharmacogenomic gene sets.
A further factor contributing to the limited value of pharmacogenomic testing is that there is limited evidence for a relation between blood concentrations and therapeutic response for many antidepressant classes, such as SSRIs. Other relevant issues are the lack of subtyping of depression in such trials and failure to control for psychotherapeutic interventions.
Conclusion
Where does this leave the clinician who wants to maximise the chance of clinical improvement for their patient with depression? I believe that universal pharmacogenomic testing (a ‘pre-emptive’ approach) is not warranted in view of the current modest evidence. The Clinical Pharmacogenetics Implementation Consortium recently made a similar recommendation.10 Further studies underway in Australia and overseas will hopefully clarify the clinical utility of such testing.11
When would I recommend pharmacogenomic testing for patients with depression? First, it would be reasonable to consider ‘reactive’ testing for patients who repeatedly experience excessive adverse effects with antidepressants at doses for which that would be surprising, suggesting the possibility of genetically determined poor metabolism. If poor metabolism is confirmed, this would guide trials of other antidepressant classes less likely to produce such effects.
Secondly, the failure to respond to different antidepressants in patients who are clinically considered likely to respond may indicate the need for reactive pharmacogenomic testing to exclude ultrarapid metabolism. The demonstration of ultrarapid metabolism would guide the clinician to trial higher doses than would usually be considered.
In conclusion, the literature and clinical experience currently suggest this limited, reactive role for pharmacogenomic testing in the management of patients with depression (summarised in the Box ). MT
COMPETING INTERESTS. Professor Mitchell has received honoraria in the past three years from Janssen (Australia) for lectures and advisory board membership and from Sanofi (Hangzhou) for lectures. He also received support for travel and accommodation from Sonic Genetics to speak at a symposium on pharmacogenetics.
References
1. Australian Bureau of Statistics. National Study of Mental Health and Wellbeing (Reference period 2020-2022; released 5/10/23). Available online at: https://www.abs.gov.au/statistics/health/mental-health/national-study-mental-health-and-wellbeing/latest-release#prevalence-of-mental-disorders (accessed November 2023).
2. Mitchell PB. Management of treatment-resistant depression. A guide for GPs. Med Today 2018; 19(11): 41-44.
3. Britt H, Miller GC, Henderson J, et al. General practice activity in Australia 2015-16. General practice series no. 40. Sydney: Sydney University Press; 2016.
4. Rush AJ, Trivedi MH, Wisniewski SR, et al. Acute and longer-term outcomes in depressed outpatients requiring one or several treatment steps: a STAR*D report. Am J Psychiatry 2006; 163: 1905-1917.
5. Campos AI, Byrne EM, Mitchell BL, et al. Impact of CYP2C19 metaboliser status on SSRI response: a retrospective study of 9500 participants of the Australian Genetics of Depression Study. Pharmacogenomics J 2022; 22: 130-135.
6. Greden JF, Parikh SV, Rothschild AJ, et al. Impact of pharmacogenomics on clinical outcomes in major depressive disorder in the GUIDED trial: a large, patient- and rater-blinded, randomized, controlled study. J Psychiatr Res 2019; 111: 59-67.
7. Oslin DW, Lynch KG, Shih MC, et al. Effect of pharmacogenomic testing for drug-gene interactions on medication selection and remission of symptoms in major depressive disorder: the PRIME Care Randomized Clinical Trial. JAMA 2022; 328: 151-161.
8. Bunka M, Wong G, Kim D, et al. Evaluating treatment outcomes in pharmacogenomic-guided care for major depression: a rapid review and meta-analysis. Psychiatry Res 2023; 321: 115102.
9. Iosifescu DV. Pharmacogenomic testing for next-step antidepressant selection: still a work in progress. JAMA 2022; 328: 146-148.
10. Bousman CA, Stevenson JM, Ramsey LB, et al. Clinical Pharmacogenetics Implementation Consortium (CPIC) guideline for CYP2D6, CYP2C19, CYP2B6, SLC6A4, and HTR2A genotypes and serotonin reuptake inhibitor antidepressants. Clin Pharmacol Ther 2023; 114: 51-68.
11. Saya S, Chondros P, Abela A, et al. The PRESIDE (PhaRmacogEnomicS In DEpression) Trial: a double-blind randomised controlled trial of pharmacogenomic-informed prescribing of antidepressants on depression outcomes in patients with major depressive disorder in primary care. Trials 2023; 24: 342.